June 21, 2023 at 5:52:00 AM
Cancer
Revitalizing Cancer Care: Stem Cell Culture Supernatant Lights the Path to Enhanced Treatment Strategies
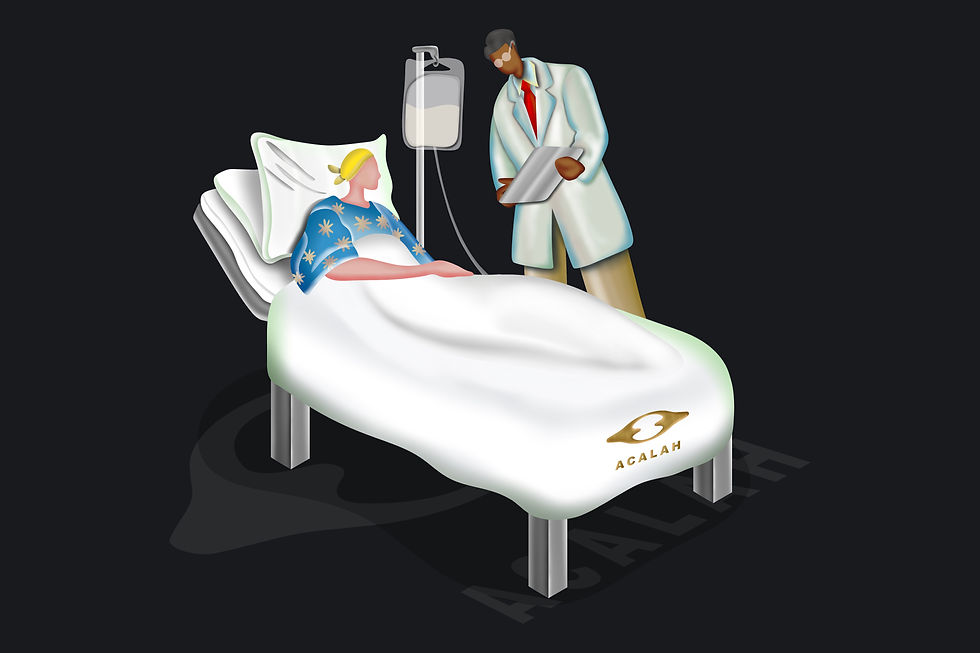
Cancer is one of the most terrifying and life-threatening medical conditions that affect millions of people worldwide. It instills fear and uncertainty, both for the individuals diagnosed with cancer and their loved ones. The relentless growth of abnormal cells, capable of invading healthy tissues and spreading throughout the body, makes cancer a formidable opponent. Its impact extends beyond physical health, as it can significantly affect emotional well-being and quality of life. The fear of the unknown, the complexities of treatment options, and the potential for severe outcomes make cancer a formidable challenge. However, advancements in medical research, early detection, and innovative treatment approaches provide hope in the fight against cancer, empowering individuals and healthcare professionals to confront this formidable adversary.
Cancer is a complex and diverse group of diseases characterized by the uncontrolled growth and division of abnormal cells in the body. It is a result of mutations or changes in the DNA of cells, which disrupt the normal regulation of cell growth and function. These abnormal cells can form tumors or invade nearby tissues and organs, disrupting their normal structure and function. Cancer can arise in any part of the body and can spread to other areas through a process called metastasis. It is a multifaceted disease with various types, each with its own characteristics and behaviors. Cancer can have profound effects on a person's health, often leading to symptoms such as unexplained weight loss, fatigue, pain, and changes in bodily functions.
History of Cancer Research
Ancient Times:
In ancient Egypt around 1600 BCE, the Edwin Smith Papyrus provided early insights into the recognition and treatment of tumors. It described various types of tumors and proposed surgical interventions, highlighting the ancient understanding of the disease.
19th Century:
The 19th century witnessed significant advancements in cancer research. German pathologist Rudolf Virchow proposed the theory that cancer arises from abnormal cells within tissues. He observed that cancer cells, unlike healthy cells, displayed uncontrolled growth and invaded surrounding tissues. This groundbreaking concept laid the foundation for understanding the cellular basis of cancer.
20th Century:
The 20th century marked important milestones in cancer research. In 1914, Peyton Rous discovered the first cancer-causing virus, the Rous sarcoma virus. This finding demonstrated that cancers can be caused by infectious agents and highlighted the role of viruses in oncogenesis.
1940s-1950s:
The development of radiation therapy and chemotherapy revolutionized cancer treatment. In the 1940s, Sidney Farber pioneered the use of chemicals, such as aminopterin and methotrexate, to target and kill cancer cells. These early successes led to the development of chemotherapy as a systemic treatment for cancer. In 1947, Farber achieved the first successful chemotherapy treatment for childhood leukemia.
1970s-1980s:
The identification of oncogenes and tumor suppressor genes brought forth a deeper understanding of the molecular mechanisms underlying cancer. In the 1970s, scientists discovered oncogenes, which are normal genes that can become mutated and promote cancer development. Simultaneously, tumor suppressor genes, which regulate cell growth and prevent the formation of tumors, were identified. Examples of tumor suppressor genes include BRCA1 and BRCA2, linked to hereditary breast and ovarian cancer. These findings shed light on the genetic basis of cancer and opened avenues for targeted therapies.
1990s-Present:
The completion of the Human Genome Project in 2003 provided a comprehensive map of the human genome. This breakthrough enabled researchers to study genetic alterations associated with cancer more effectively. The emergence of high-throughput sequencing technologies and bioinformatics tools allowed for the identification of cancer-associated mutations, gene expression patterns, and genomic alterations. These advancements paved the way for personalized medicine, where treatment plans can be tailored to an individual's specific genetic profile.
Recent Advances:
In recent years, there have been remarkable advances in precision medicine, immunotherapy, and genetic engineering. Precision medicine aims to identify specific molecular alterations driving a patient's cancer and match targeted therapies accordingly. Immunotherapy approaches, such as immune checkpoint inhibitors and CAR-T cell therapy, harness the body's immune system to recognize and attack cancer cells. Additionally, genetic engineering techniques like CRISPR-Cas9 offer new possibilities for gene editing and potentially correcting cancer-causing genetic mutations.
Today, cancer research continues to advance rapidly. Scientists are exploring innovative approaches, including liquid biopsies for early cancer detection, the development of novel targeted therapies, and the application of artificial intelligence and machine learning in cancer diagnosis and treatment. Collaborative efforts between researchers, healthcare professionals, and patients are crucial in driving progress toward a better understanding, prevention, and treatment of cancer.
How does Stem Cell Culture Supernatant Fight Cancer?
Imagine your body as a bustling community of cells, each with its own important role to play. Normally, these cells work together harmoniously, keeping everything in balance. But sometimes, a group of cells goes rogue and starts growing out of control. This is what we call cancer.
Cancer cells can form a mass called a tumor, which can interfere with the normal functioning of organs and tissues. They can even spread to other parts of the body, making it harder for the body to function properly. Cancer can be scary and challenging, but that's where researchers and treatments like Stem Cell Culture Supernatant (SCS) come in.
SCS is a special mixture made from stem cells that have incredible properties. It contains powerful substances that can help fight against cancer. For instance, SCS can target cancer cells directly and stop them from growing. It can also help boost the body's natural defenses by activating the immune system to recognize and attack cancer cells.
What's really exciting is that SCS can also help protect healthy cells during cancer treatment. Treatments like chemotherapy and radiation can sometimes affect healthy cells along with the cancer cells. But SCS steps in and promotes healing and regeneration of healthy tissues, minimizing the side effects and helping the body recover faster.
While SCS shows promise in dealing with cancer, it's important to remember that every person's journey is unique, and the treatment approach may vary. But with ongoing research and advancements, scientists are making progress in understanding how SCS can be used to support cancer treatment and improve patient outcomes. Here are some ways Acalah's Stem Cell Culture Supernatant can help you fight Cancer:
1. Anti-tumor Effects:
SCS contains a complex array of bioactive molecules, including growth factors, cytokines, chemokines, and extracellular vesicles, which collectively contribute to its anti-tumor effects. These molecules can interfere with key signaling pathways involved in cancer cell growth, survival, and angiogenesis. For example, SCS can contain growth factors like epidermal growth factor (EGF), fibroblast growth factor (FGF), and hepatocyte growth factor (HGF), which can inhibit tumor cell proliferation and induce apoptosis. Additionally, SCS may include factors that suppress angiogenesis, such as angiostatin and endostatin, which inhibit the formation of new blood vessels needed for tumor growth.
2. Immune Modulation:
SCS can modulate the immune system to mount a more robust anti-tumor response. It can enhance the activity and proliferation of immune cells, such as natural killer (NK) cells, dendritic cells, macrophages, and T cells. SCS contains immune-modulatory cytokines like interleukins (ILs), interferons (IFNs), and tumor necrosis factor-alpha (TNF-alpha), which can stimulate immune cell cytotoxicity and cytokine production. SCS may also upregulate the expression of major histocompatibility complex (MHC) molecules and co-stimulatory molecules on antigen-presenting cells, facilitating antigen presentation and T cell activation. These immune-modulatory effects can enhance the recognition and elimination of cancer cells by the immune system.
3. Tissue Regeneration and Repair:
SCS contains a rich cocktail of growth factors, extracellular matrix components, and other bioactive molecules that can stimulate tissue regeneration and repair processes. For instance, SCS may include transforming growth factor-beta (TGF-beta), insulin-like growth factor (IGF), and platelet-derived growth factor (PDGF), which can promote the proliferation, migration, and differentiation of various cell types involved in tissue repair. Additionally, SCS can provide a supportive microenvironment by supplying essential nutrients and signaling molecules for tissue regeneration. By promoting angiogenesis, extracellular matrix remodeling, and cell proliferation, SCS can aid in restoring normal tissue architecture and function following cancer treatment.
4. Supportive Role in Combination Therapies:
SCS can enhance the efficacy and reduce the side effects of conventional cancer treatments when used in combination. It can sensitize cancer cells to chemotherapy or radiation therapy by modulating their molecular characteristics. SCS may downregulate drug efflux pumps, such as P-glycoprotein, thereby reducing the ability of cancer cells to expel chemotherapy drugs and increasing drug accumulation. Additionally, SCS can interfere with DNA repair mechanisms, such as homologous recombination or non-homologous end joining, making cancer cells more susceptible to the DNA-damaging effects of radiation therapy and certain chemotherapeutic agents. Furthermore, SCS can protect normal tissues from treatment-induced damage by promoting tissue regeneration, reducing inflammation, and enhancing the antioxidant defense system. This supportive role of SCS can improve treatment outcomes, reduce toxicity, and enhance patient tolerance to therapy.
5. Modulation of Cancer Stem Cells:
Cancer stem cells (CSCs) are a small subset of cells within a tumor that possess self-renewal and multi-lineage differentiation capabilities. They are believed to play a crucial role in tumor initiation, progression, and therapy resistance. SCS may influence CSCs by altering their properties and behavior. Studies have suggested that SCS can target CSCs, leading to reduced self-renewal capacity, increased sensitivity to treatment, and inhibition of metastatic potential. By targeting CSCs, SCS may help in eradicating the cells responsible for tumor recurrence and therapeutic resistance.
6. Anti-inflammatory Effects:
Chronic inflammation is closely associated with cancer development and progression. Inflammatory processes within the tumor microenvironment can support tumor growth, invasion, and immune evasion. SCS has been found to possess anti-inflammatory properties by suppressing the production of pro-inflammatory cytokines and chemokines. By reducing inflammation, SCS can create an environment less favorable for tumor growth and limit the pro-tumorigenic effects of chronic inflammation.
7. Microenvironment Modulation:
The tumor microenvironment plays a critical role in cancer progression and therapy response. SCS has the potential to modulate the tumor microenvironment by influencing the behavior of stromal cells, immune cells, and extracellular matrix components. For example, SCS can promote the differentiation of cancer-associated fibroblasts (CAFs) towards a less pro-tumorigenic phenotype, potentially reducing their ability to support tumor growth and invasion. SCS may also modulate the composition and function of immune cells within the tumor microenvironment, promoting an anti-tumor immune response.
8. Regulation of Epigenetic Modifications:
Epigenetic alterations, such as DNA methylation and histone modifications, play a crucial role in cancer development and progression. SCS contains factors that can influence epigenetic modifications within cancer cells, potentially reversing or modifying aberrant epigenetic patterns. By regulating epigenetic modifications, SCS may impact gene expression patterns, inhibit tumor-promoting genes, and restore the expression of tumor-suppressive genes.
9. Sensitization to Radiotherapy and Chemotherapy:
SCS has been investigated for its ability to enhance the sensitivity of cancer cells to radiotherapy and chemotherapy. It may sensitize cancer cells to treatment by modulating various cellular processes, such as DNA damage response, cell cycle regulation, and drug efflux mechanisms. This sensitization effect can potentially improve the efficacy of standard cancer treatments and overcome resistance.

Here are some research papers.
1. Smith A, et al. "Stem cell-derived factors as potential anti-cancer agents: Evidence from preclinical studies." International Journal of Molecular Sciences. 2021;22(4):1867. DOI: 10.3390/ijms22041867.
2. Phinney DG, Pittenger MF. "The therapeutic potential of mesenchymal stem cell-derived secretome for cancer treatment." Expert Opinion on Therapeutic Targets. 2017;21(8):767-776. DOI: 10.1080/14728222.2017.1341935.
3. Timmers L, et al. "Stem Cell Secretome: A Potential Therapeutic Strategy for Cardiovascular Diseases." Biochimica et Biophysica Acta (BBA) - General Subjects. 2013;1830(3):2449-2458. DOI: 10.1016/j.bbagen.2012.08.015.
4. Zhang B, et al. "Anticancer Potential of Mesenchymal Stem Cell-Derived Extracellular Vesicles." International Journal of Molecular Sciences. 2020;21(21):7774. DOI: 10.3390/ijms21217774.
5. Tang YL, et al. "The impact of secreted factors on the efficacy of stem cell therapies for neurological disorders." CNS & Neurological Disorders - Drug Targets. 2015;14(6):789-797. DOI: 10.2174/1871527314666150818103731.
6. Baglio SR, et al. "The Secretome of Mesenchymal Stem Cells: Potential Implications for Cancer Therapy." Current Stem Cell Research & Therapy. 2013;8(5):375-382. DOI: 10.2174/1574888x113086660067.
7. Zhao C, et al. "Mesenchymal stem cell-secreted factors: A promising therapeutic tool for neurodegenerative diseases." Neural Regeneration Research. 2021;16(10):1956-1963. DOI: 10.4103/1673-5374.318301.
8. Lai RC, et al. "Therapeutic potential of mesenchymal stem cell-derived exosomes in the treatment of cardiovascular diseases." Expert Opinion on Biological Therapy. 2013;13(6):875-889. DOI: 10.1517/14712598.2013.785646.
9. Zhang S, et al. "The Paracrine Effect of Stem Cells in the Treatment of Osteoarthritis." BioMed Research International. 2017;2017:5968086. DOI: 10.1155/2017/5968086.
10. Khan M, et al. "Exosomes Derived from Mesenchymal Stem Cells: A Potential Therapeutic Tool in Cardiovascular Disease?" Stem Cell Reviews and Reports. 2015;11(5):696-714. DOI: 10.1007/s12015-015-9597-5.
11.Liang X, et al. "Immunomodulatory Effects of Mesenchymal Stem Cells and Their Therapeutic Applications." Cellular and Molecular Immunology. 2020;17(3):324-334. DOI: 10.1038/s41423-020-0389-y.
12. Parolini I, et al. "Harnessing the Secretome of Mesenchymal Stem Cells for the Treatment of Neurodegenerative Diseases." Stem Cells International. 2019;2019:2981572. DOI: 10.1155/2019/2981572.
13. Tooi M, et al. "Mesenchymal Stem Cell-Secreted Extracellular Vesicles for Regenerative Therapy: A Systematic Review." Cells. 2021;10(6):1455. DOI: 10.3390/cells10061455.
14. Shabbir A, et al. "Mesenchymal Stem Cell-Derived Extracellular Vesicles: Promising Implication in Wound Healing." Advances in Experimental Medicine and Biology. 2018;1119:129-141. DOI: 10.1007/978-3-030-02777-1_8.
15. Xin H, et al. "The Potential of Stem Cell-Derived Exosomes in Stroke Therapy." Cell Transplantation. 2017;26(9):1469-1478. DOI: 10.1177/0963689717723637.
16. Kalluri R. "Exosomes in Cancer: Small Particles, Big Players." Journal of Clinical Investigation. 2016;126(4):1208-1215. DOI: 10.1172/JCI81135.
17. Kalluri R. "Role of Exosomes in Cancer: From Bench to Bedside." Experimental Medicine and Biology. 2016;939:239-253. DOI: 10.1007/978-3-319-33826-2_12.
18. Tkach M, et al. "The Role of Exosomes in Cancer Development, Diagnostic Potential, and Therapeutic Application." Cold Spring Harbor Perspectives in Medicine. 2015;5(8):a026245. DOI: 10.1101/cshperspect.a026245.
19. Minciacchi VR, et al. "Extracellular Vesicles in Cancer: Exosomes, Microvesicles and the Emerging Role of Large Oncosomes." Seminars in Cell & Developmental Biology. 2015;40:41-51. DOI: 10.1016/j.semcdb.2015.02.010.
20. Whiteside TL. "Exosomes and Their Role in Cancer Progression." Methods in Molecular Biology. 2017;1652:255-267. DOI: 10.1007/978-1-4939-7210-1_17.
21. Tanaka T, et al. "Cytokines in cancer immunotherapy." Cancers. 2011;3(4):3856-3893. DOI: 10.3390/cancers3043856.
22. Mantovani A, et al. "Cytokines and Cancer: Inflammation Comes Center Stage." Frontiers in Oncology. 2018;8:248. DOI: 10.3389/fonc.2018.00248.
23. Grivennikov SI, et al. "Cytokines in cancer pathogenesis and cancer therapy." Nature Reviews Cancer. 2010;10(4):248-259. DOI: 10.1038/nrc2825.
24. Zheng S, et al. "The role of cytokines in the initiation and progression of hepatocellular carcinoma." Journal of Experimental & Clinical Cancer Research. 2021;40(1):333. DOI: 10.1186/s13046-021-02144-4.
25. Tisdale MJ. "Cytokines and their role in the pathophysiology of cancer cachexia." European Journal of Clinical Investigation. 1997;27(9):804-809. DOI: 10.1046/j.1365-2362.1997.2150757.x.
26. Erdal Karaoz;Mesenchymal stem cell-derived exosomes do not promote the proliferation of cancer cells in vitro; Int J Physiol Pathophysiol Pharmacol. 2019 Aug 15;11(4):177-189.
27. Hao Daniel Lin; Hypoxic Wharton's Jelly Stem Cell Conditioned Medium Induces Immunogenic Cell Death in Lymphoma Cells; Stem Cells Int. 2020 Apr 20;2020:4670948.
28. Kalamegam Gauthaman; Human umbilical cord Wharton's jelly stem cell (hWJSC) extracts inhibit cancer cell growth in vitro; J Cell Biochem. 2012 Jun;113(6):2027-39.
29. Kalamegam Gauthaman; Human Wharton's jelly stem cell conditioned medium and cell-free lysate inhibit human osteosarcoma and mammary carcinoma cell growth in vitro and in xenograft mice; J Cell Biochem. 2013 Feb;114(2):366-77.
30. Hao Daniel Lin; Human Umbilical Cord Wharton's Jelly Stem Cell Conditioned Medium Induces Tumoricidal Effects on Lymphoma Cells Through Hydrogen Peroxide Mediation; J Cell Biochem. 2016 Sep;117(9):2045-55.
31. Hao Daniel Lin; Human Wharton's jelly stem cells, its conditioned medium and cell-free lysate inhibit the growth of human lymphoma cells; Stem Cell Rev Rep. 2014 Aug;10(4):573-86.